Fabric and foil membranes offer architects unique design opportunities.
By Nicholas Goldsmith, FAIA LEED AP
Looking at a historical perspective on the evolution of architecture — from the massive pyramids of Egypt to the framed structures of Greek and Roman construction, to the lighter Gothic vaulting and eventually modern architecture of the 20th century — there is a continuous, almost linear progression from solid mass to diaphanous skins of glass and steel.
A record of earlier brick and wood structures in Egypt gave way to the presence of the earliest stone architecture some 5,000 years ago, and because of the Egyptian obsession with the afterworld and permanence, a culture of stone was created.
As culture shifted from Egypt to Greece and Rome, stone continued to be used, but the Greek and Roman temples used larger spans for their columns and less material overall. The classical three orders started with the Doric, to Ionic to Corinthian — each becoming lighter in profile. With the advent of Byzantine and Romanesque churches, the weight to span decreased; and when the Gothic style was developed in northern Europe in the 12th century, this decrease in weight to span accelerated with the introduction of flying buttresses that allowed glass curtain walls reflecting its metaphysical attraction to light appear seemingly self-supporting in heights of up to 150 feet.
In the 20th century, a movement from mass to membrane increased in speed with the introduction of steel framing, lattice trusses and new developments in glass technology. By the beginning of this century, glass had become the material of preference. Curtain walls using truss framing, diagrids, glass mullions and cable systems all became common architectural practice. Corporations used glass towers as a symbol of prestige and transparency. Today, lighter and lighter building technologies include structural membranes composed of fabric and foils. It is interesting to note that two of the leading high-tech companies of world, Google and Apple, presently are developing glass and ethylene tetrafluoroethylene (ETFE) foil skins as elements of their new corporate headquarters.
In short, looking back over the last 4,000 years of architecture, one can see a linear evolution from the mass of the pyramids to the membranes of tomorrow. Mass to membrane is a human architectural journey — can we use less material, be more sustainable, and help reduce the carbon footprint on the planet called earth?
Fabric, foils and other membranes are materials well suited for creating building skins. They are curved for strength, provide waterproofing and insulation, can be multi-layered and can create complex surfaces with minimal seaming. Fabric skins have been around since the beginning of time as seen in yurts and leather tents of ancient civilizations. But with today’s new composite materials, computational analysis and digital patterning, membranes have developed into a new option as permanent building skins.
Building Skins
To shed light on the new building skins of tomorrow and new approaches to building skins, step back and look at human skin. There are three layers of skin: the epidermis, the dermis and the hypodermis. The outer epidermis layer contains the sweat pores and hair shafts; the middle dermis layer contains connective tissues, hair follicles, sweat glands and some muscles; and the inner hypodermis layer contains veins and arteries as well as connective tissues that tie the layers together.
This approach of multiple integrated layers all performing different functions creates a skin that gives the body a waterproof cover, keeps out disease and protects against sunlight. When the approach of multiple integrated layers is applied to architectural membrane structures, the performance requirements of the building skin first need to be understood.
Not only is human skin sensitive, sending the brain information about pressure and temperature, but it heals efficiently to maintain a protective barrier. Combining these two features into one man-made material has been the focus of Stanford University chemical engineering professor Zhenan Bao and her team. The team has succeed in making a man-made skin that is not only sensitive to touch, but also is capable of healing itself quickly and repeatedly at room temperature, which has significant implications for biomedical textile markets. The researchers succeeded by combining two materials — a plastic polymer with self-healing ability and the conductive metal.
Today, enormous growth is observed in the wearables or smart garments market. These technologies use intelligence pathways in the fibers of the materials to create e-textiles. Socks, sports bras, T-shirts with heart sensors, and casts with currents for quickly knitting bones together are now all available products. As this market develops, spin-off technologies likely will have an impact building skin technologies.
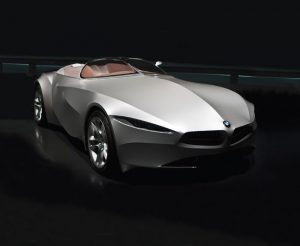
A few years ago, New York City-based FTL Design Engineering Studio assisted Klaudia Kruse and the innovation team at BMW to develop a stretch fabric body for a concept car named the Gina after the famous Italian actress Gina Lollobrigida. The stretch fabric approach was based on a lightweight aluminum frame with a fabric skin that can open and close as a complete shape (See Figure 1). This is a fabric skin approach to industrial design that is in its early stages of development, but again asks the question of how to get more when using less material.
Structure
There are three basic types of building skin structures — frame supported membranes, cable and strut supported membranes, and either air-supported or air-inflated pneumatic membranes. Each one of these systems requires the skin to be prestressed to develop strength, and requires form-finding to develop relaxed 3-D — either synclastic or anticlastic — surfaces that are in equilibrium. Ideally, they have equal tension and are considered minimal surfaces, but often for spatial reasons they have different tensions in both opposing primary directions. Whether computer programs that utilize a force density approach or a dynamic relaxation approach are used for form finding and analysis, finite element analysis is required to create balanced structural skins that can resist downward and uplift pressures.
Form
Prestressed membranes take either saddle surfaces — hyperbolic paraboloids — toroid or doughnut shapes; and surfaces of revolution — either hyperboloid or toroid surfaces. In complex building skins, these basic building block elements are developed into multiple panels where the different surfaces are bounded by ridges and valleys to create complex geometries. The intersection of the ridge lines and valley lines become 3-D linear elements that are described by physical modeling and form-finding geometries.
Acoustics
There are many different approaches to generating form including considering acoustics, site constraints, sun angles and wind. Using acoustics, one starts with a ray diagram to generate reflective surfaces that both blend and reflect sound waves. Depending on whether the structure is used for pop music or classical music determines the type of surface required for performances. Conoid shapes and synclastic shapes tend to trap sound, so most performance pavilions use a series of hyperbolic surfaces to create a rich interior experience.
A project in Sun Valley Idaho completed by FTL Design Engineering Studio in 2008 uses acoustic reflection and the site’s constraints as the form generator for the music pavilion. The site was a landscape in the Rocky Mountains at a famous ski resort frequented by Hollywood celebrities. FTL’s approach to the landscape was to develop a series of retaining walls that rose out of the land, came together to create a musical space and then faded back into the ground. The music season lasted only through the summer months, but the winter snow load criteria was more than 100 pounds per square foot (lb/ft2) with drifts of up to 200 lb/ft2. FTL understood the symphony wanted a structure that combined the seasonal and ephemeral quality of a summer tent with a more permanent facility that could accommodate the theatrical and acoustic requirements. FTL realized the local snow load requirements would not allow for a permanent fabric structure. The eventual design features a steel cable net and uses a rigid wood material that can handle high vertical loads. When developing the idea, FTL felt that the wood was very much in keeping with the materials near the site and tied into the surrounding buildings. A copper-top roofing maintained the differential in slope and added another earthy touch to the ephemeral cable structure. The audience area remains covered with a tensile fabric skin that is demounted each fall prior to the winter.
Musically, the wood roof allows for a rich sound that is augmented by a series of acoustical reflector panels suspended below the cable net, which can be fine-tuned for different types of music. In this case, a hybrid solution of the cable net and tensioned fabric solved the diverse set of design challenges.
Shading And Insulation
One of the quintessential qualities of tensile structures allows their use as shading elements. Since ancient times, membranes have been used for this purpose — whether it is the “vela” shading Roman coliseums or the Bedouin black goat hair tents of Arabia or the “toldos” covering the streets in southern Spain. With fabrics that allow anywhere from zero to 40-percent translucencies, the level of shade is controllable based on different climates and regions of the world. Based on a classification of different climates such as the 19th century professor Koeppen’s climate categorization based on plant life around the planet, one can apply both shading and insulation to lightweight structures. The strategies for membranes in cold moist climates such as northern Europe are considerably different than hot arid climates such as the American southwest or the hot and moist climates of Central America.
Technologies such as foil pillow structures make sense in the cold, moist climates; while in hot and arid climates, mesh fabrics often are used; and in hot and moist climates, solid fabrics that provide for air movement and passive cooling are used.
FTL had to consider a northern climate when designing an entryway for the Empire City Casino in Yonkers, N.Y. The design firm developed an ETFE foil pillow system supported by a steel grid shell structure that acts as an entry to the casino and also provides rain and sun protection for up to 30 cars. The design also acts as a lighting element and an icon for the casino. FTL developed a toroid shape for the grid shell, but also looked at funicular shapes to minimize steel weights. The ETFE pillows allow for transparencies up to 98 percent, and are a soft alternative to glass roofs and facades.
Energy Generation
By their nature, membrane structures use large unsupported spans that can be modified with the application of solar thin film technologies to generate power to the space inside. In addition, tensioned membranes can serve as shrouds for wind collection turbines where minimizing turbulence allows the turbines to work efficiently at lower wind speeds. Since the first application of solar panels that FTL pioneered in 1998 at the National Design Museum in New York, the company has worked with amorphous silicone and copper indium gallium selenide (CIGS) technologies to develop alternative methods of power generation using building skins. Generating only approximately 5 watts per square foot, the method is still relatively inefficient. But because the roof area of the building skin is generally large, the inefficiency of the thin film technology is offset by its cost effective size.
Lighting
Several basic forms of illumination exist in nature— illuminant, illumination, film mode, surface mode and volume mode. Membranes can make use of surface and volume natural lighting modes. Using artificial lighting through the volume light mode creates glowing volumes of form and space. FTL’s recent project for Phoenix-based Arizona State University modeled computer lighting to create glowing volumetric light using high-intensity discharge fixtures for the main surfaces and programmable light emitting diode lights to highlight the steelwork.
Water Collection
Water collection always has been an important function of roofing in dry climates since Roman times. But with today’s added interest in sustainable development and storm water collection, interest in collecting storm water in cisterns for reuse has increased. Membrane structures are ideal collectors of rainwater, and the shape of the roof can be manipulated to become a water feature of the building.
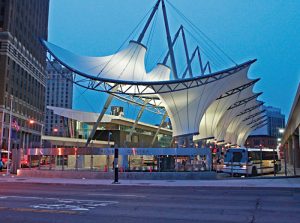
In 2009, FTL completed the Rosa Parks Transit Center in downtown Detroit for the city (See Figure 2). The goal was to unify six bus waiting areas and connect them to the “People Mover” — an elevated rail system that serves the City. The design firm developed a 6,000-square-meter roof under which 13 busses can load and unload passengers. Because of the unique urban site and the elevated tracks of the rail system, foundation placement was very limited. However, the design was required to cover the street area up to the elevated tracks. FTL’s solution to this dilemma was to create a series of seven A-frame masts that use a tensegrity cable system to suspend eight curved trusses. By cantilevering the trusses out over the road, the bus area was covered. The section of trusses with A-frames creates peaks and low points, which provides curvature to the fabric, but also creates the opportunity to collect water from the roof, which is stored in a cistern below grade. The edge of the low point has a large gutter and feeder pipes that allow the water to pour into a landscaped element with a catch basin at grade. The water collection areas are surrounded by built-in seating for waiting passengers. The stored water is used for irrigation for plants and landscaping on site.
Future Outlook
There are many different elements required for building skins depending on the location they will be used. The task ahead focuses on integrating these different elements into multi-skin solutions, much like human skin, to offer numerous functions. The next steps in the evolution of membrane structures will be to layer integrated systems that, when connected, will transform the notion of building facades into a porous multifunctional membrane reflecting the natural world.
Editor’s Note: Nicholas Goldsmith, FAIA LEED AP, is senior principal at New York City-based FTL Design Engineering Studio; and a fellow of the American Institute of Architects, International Association for shell and spatial structures, as well as the former chair of the Lightweight Structures Association. This article is based on Goldsmith’s presentation given at the 2015 Textile World Innovation Forum.
September/October 2016