S
tudies in the area of “electronic textiles” or “electrotextiles” have captured
researchers’ attention worldwide. Recently, many reports and papers have been published, and
conferences and symposiums held covering this fast-growing area of research and development.
The reason for such interest is the potential to develop smart fabrics that can sense,
respond and adjust to stimuli such as pressure, temperature or electrical charge. Thermal clothing
such as blankets and jackets that protect humans, pets and animals from cold weather, musical
jackets and a flexible foldable computer keyboard are some electrotextile products that are
commercially available today. But these are only the beginning. Material scientists, textile
engineers and technologists, computer scientists, and electrical engineers are working on combining
electronics and textile structures to come up with new products that are flexible, conformable and
lightweight; and which potentially may be produced at the speed of current traditional textile
structure manufacturing.
Research and development targets civilian and military applications such as: woven antennae;
acoustic array – a woven fabric circuit combined with an acoustic sensor that locates sources of
sound, such as gun shots or enemy vehicles; active woven fabrics with electroactive constituents to
improve the drop precision of parafoils; formation of transistors on flexible substrates, such as
thin ribbon yarns; yarn batteries and flexible and conformable solar cells to harvest and provide
electrical power to electrotextiles; and flexible textile circuitry used for fashion and design –
for example, in fabrics that can change color on demand. It is expected that electrotextile
products will find their way to new markets such as healthcare, entertainment, safety, homeland
security, computation, communication, thermal uses, protective clothing, wearable electronics and
fashion, as well as energy harvesting from tensioned structures and other large-area fabrics.
New advances in weaving technologies have led to the development of high-speed, highly
automated weaving machines. This combination and the ordered structure of woven fabrics provide new
opportunities for the weaving industry to produce woven fabric circuits and electrotextiles at high
speed.
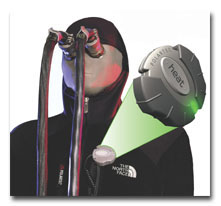
Lawrence, Mass.-based Malden Mills Industries Inc.’s Polartec®Heat™ technology —
available in this North Face MET5 jacket — features heated panels connected to a lithium
battery-powered controller to deliver three modes of user-adjustable warmth. The jacket is shown
undergoing Thermal Mannequin Testing for insulation and wicking properties at the US Army Natick
Soldier Systems Center, Natick, Mass.
Relevance Of Woven Structures
Due to their excellent mechanical and physical properties, woven fabrics have established
themselves in many different end-use products. Today, woven fabrics are used in countless civilian
and military applications such as apparel, upholstery, curtains, seat covers, seat belts, rugs,
wall covers, parachutes, weather balloons, tents, vehicle covers, and air-supported and tensioned
structures. They are the choice for many applications for various reasons including: high
uniformity; high strength; high tear and abrasion resistance; controllable air permeability;
dimensional stability; durability; flexibility; conformability; recovery from relatively high
stresses; and light weight.
In addition, woven fabric structures can provide a complex network that can be used as
elaborate electric circuits with numerous electrically conducting and non-conducting constituents,
and can be structured to have multiple layers and spaces to accommodate electronic devices. For
example, rigid printed circuit boards are structured with wiring layers separated by insulating
layers with vias connecting power, ground lines and wiring of different layers to process/transfer
signals. These boards, which are fabricated using the slow process of photolithography, can
potentially be replaced with flexible, stitched, multi-layered woven fabric structures that can be
produced at speeds of 40 to 100 square meters per hour depending on weaving speed, pick density and
weaving machine width
(See Figure 1). The stitches in such fabrics constitute the vias.
Currently, there are many commercial electrically conducting yarns made from metals or from
polymers coated with metals. Some examples of these are short-staple and continuous-filament steel
yarns, copper yarns, and silver-coated nylon yarns. These yarns are made from very thin fibers or
filaments that make them feel and behave like textile yarns.
Current studies indicate that researchers will meet the challenge and will succeed in
producing polymeric yarns with electrically conducting cores, solar-cell yarns, battery yarns and
electronic devices with sub-micron thicknesses built – or extruded as continuous filament – on
yarns. Furthermore, it is believed that these yarns can be produced in large quantities and may be
available on package forms similar to those for traditional textile yarns. When these
products become a reality, complex multifunctional “electrowoven” structures – or electronic woven
structures – may be produced at high speeds. While new advances in weaving would be assets in this
vein, many other challenging developments are needed as well.
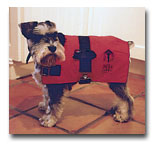
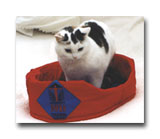
Electro-conductive textile (E-CT) products, such as the Infra-Therm Heated Dog Jacket and
Infra-Therm Rug Heated Cat Basket, from England-based Gorix Ltd. have low ohmic resistance and
become hot when excited by low-voltage electricity.
Automatic Formation Of Woven Circuits
Formation of woven circuits requires the interconnecting or welding of two or more
orthogonal yarns at selected crossover points. Because yarns in woven fabrics run from selvage to
selvage, or continuously along the fabric length, cutting of yarns may be required depending on
circuit design. The formation of interconnects and disconnects, or cutting, of today’s electrowoven
products is conducted manually post weaving. While today’s electrowoven products are simple, future
products will demand more complex circuits. To avoid human errors and provide affordable products,
automatic formation of interconnects/disconnects is essential.
Several interconnect and disconnect methods have been identified that could be automated.
Interconnect methods include resistance-welding using microprobes and air-splicing, which is used
successfully in the joining of two yarn ends in automatic yarn winding. Disconnect techniques
include cutting with microcutters and controlling the welding parameters during resistance-welding
to cut and interconnect at the same time.
To automate the formation of interconnects/disconnects, weaving machines with small robotic
devices to manipulate an air splicer, a microcutter and a resistance-welding device, or equivalent
devices, need to be developed. Existing automatic filling repair mechanisms may be modified to
include the interconnect/disconnect devices. Optical sensors may be needed to locate the positions
of interconnects and disconnects. The need for optical sensors could be eliminated by the use of
jacquard weaving, in which every warp and filling yarn location is identified – and, hence, every
crossover point location is known.
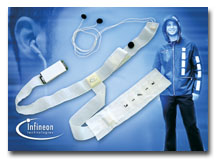
Infineon Technologies AG, Germany, has developed a prototype MP3 player that can be sewn
directly into clothing. The player consists of a chip, a removable battery/data storage card,
flexible keyboard, and includes an earpiece for listening to songs.
Formation Of Electrowoven
Structures At High Speeds
Ongoing research shows that producers of electrowoven structures will have to deal with
different yarns, including traditional textile yarns, conducting metallic or a combination of
polymeric/metallic yarns, battery yarns, solar-cell yarns, and yarns that have built-in electronic
devices. These yarns are expected to be of different physical and mechanical properties. Moreover,
yarns with devices may be in ribbon form, and yarn orientation is necessary. In such cases,
twisting of ribbon yarns must be avoided during weaving.
Recent advances in weaving technology meet some of these challenges. Variable-speed weaving
is an essential requirement for handling different filling yarns. In this case, since electrowoven
structures demand high quality and precise spacing of filling, the machine can run at high speeds
when weaving strong yarns and can be slowed down when weaving weaker yarns to avoid stops and
potential quality problems caused by stops. A filling selection mechanism of up to 12 colors is now
available, which provides the versatility required for electrowoven fabrics.
“Electroweaving” is expected to require modification of existing filling yarn feeders or
development of new filling feeders. It may be necessary to create filling feeders with guiding and
tensioning systems to make them suitable for feeding and maintaining orientation of ribbon yarns.
Needles and devices to introduce such filling yarns to the filling insertion may need to be
modified and/or developed. The newly developed electronic yarns may require development of new
filling feeders because of their differences in properties as compared to traditional yarns.
The same discussion can be made regarding electronic warp yarns. Because their physical and
mechanical properties are expected to be different from traditional warp yarns, differential in
yarn take-up during weaving is expected. This mandates feeding such yarns from a creel or separate
warp beam. A creel that has rotating packages and suitable guiding elements is a must to feed
electronic ribbon warp yarns to avoid yarn twisting and maintain orientation. One scenario may
require setting the traditional warp yarns on the main warp beam and feeding different electronic
yarns from a creel. The creel in this case may require rotating packages and individual automatic
setting of yarn tension. However, this type of creel does not exist today.
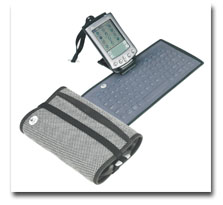
Logitech Inc., Fremont, Calif., manufactures a soft-touch KeyCase™ keyboard that can wrap
around a personal digital assistant (PDA) for maximum protection and compact
storage.
Filling Insertion System
For Electrowoven Fabrics
Air-jet and projectile filling insertion systems are obviously suitable for simple
electrowoven fabrics. The rapier filling insertion system seems to be the ultimate candidate for
weaving current and future electrowoven fabrics. However, novel rapier heads that are capable of
handling a broad range of traditional yarns and electronic yarns (especially ribbon yarns) are
required.
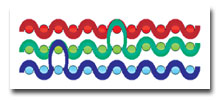
Figure 1: Illustration of a flexible, stitched, multi-layered woven structure
Versatility And Quality
The production of electrowoven fabrics will require short runs and a totally versatile
operation to produce different circuits of varying complexity and to manufacture fabrics with tight
specifications and high quality.
Short runs and versatile weaving operations have been made possible by many developments
that have taken place in recent years. These include quick style change, automatic pattern change
and variable pick density. Weaving machines today are modular, and weavers have full control over
their machines through digital interface. It is now possible to send woven fabric designs from a
CAD system to a weaving machine through a local area network (LAN) or from anywhere in the world
through the Internet.
Electrowoven fabrics must be of high quality and precision. Start-mark prevention mechanisms
and on-line fabric inspection are systems that would help to achieve high quality and precision.
Current start-mark prevention systems however, require operator intervention to set the system for
new styles. This is a challenging task for the operator when dealing with electrotextile fabrics of
short runs and variable pick density. Weaving machine manufacturers will have to create smarter
start-mark prevention systems that do not need operator intervention.
Weaving technologies are versatile and could automatically form complex, sophisticated,
high-quality electrotextiles with tight specifications at high speeds. They could allow weavers to
capture a large share of the present and future electrotextile markets. While there are many recent
weaving advances that are relevant to the production of electrowoven fabrics, there are many
developments that still need to be done.
Editor’s Note: Abdelfattah M. Seyam is a professor in the department of textiles and apparel,
technology and management at North Carolina State University’s (NCSU) College of Textiles, Raleigh,
N.C. He obtained a B.S. and M.S. in textile engineering from Alexandria University, Alexandria,
Egypt, and also holds a Ph.D. in fiber and polymer science from NCSU.