Abstract
This paper provides the latest insights into emerging technology to enhance wearability of
e-textiles and smart clothing by reviewing the cutting-edge researches and development.
Misconception of wearability in smart wearable systems is pointed out, and attachable technological
components are suggested as one of the best known solutions. Such components allow the most fragile
technologies to be protected or removed depending on the environmental changes or users’
preferences.
Based on the concept of attachable electronics, the textile transmission system is introduced
in which transmission lines and connectors are integrated to the fabric. The textile transmission
system is designed to connect attachable electronic devices by supplying power and transferring
data signals. Relevant fabrication issues — such as design of conductive yarns, integration or
interconnection methods, and connectors — are studied.
Introduction
Although electro-textiles attract a great deal of interest in relevant industries and
academia, they do not have any official definition. Electro-textiles, known as e-textiles, refer to
fabrics that can electrically function as electronics and physically behave as textiles. The
prominent application of e-textiles is smart clothing. Generally, “smart clothing system” refers to
a new garment feature that can provide interactive reactions by sensing signals, processing
information and actuating the responses.
Poorly matched to the name of clothing, however, current smart clothing systems are not
practically wearable. The clothing is wired with cables crisscrossing all over and batteries or
hard electronic devices sticking out. The problem of wearability is related to a misconception of
the word “wearable”. Initiated in the concept of a wearable computer, a wearable system was
originally understood as the use of the human body or the piece of clothing to support
technological devices. It was much later on that the concept of wearability became more practical,
addressing issues of comfort, light weight, breathability, and care and maintenance.
Having considered the true wearability, the most feasible way to wear complicated electronics
or computers at this point is to use attachable electronic components
(See Figure 1). The clothing itself carries only transmission lines and connectors so that
clothing can be flexible and washable enough to be wearable. The attachable electronic system
consists of textile transmission lines and connectors. Structures and technologies for textile
transmission lines, interconnection methods, and connectors would be explored from a textile
perspective.
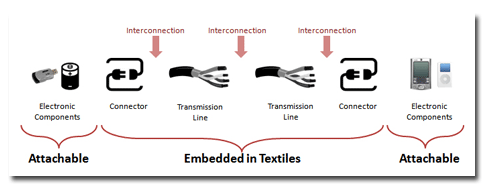
Figure 1:
Concept Of Attachable Electronics
Textile Transmission Line
A textile transmission line consists of conductive yarns integrated into a flexible textile
base. Conductive yarns are either pure metal yarns or composites of metals and non-conductive
textile materials that help improve mechanical properties. In order to produce a successful textile
transmission line, the best mix of conductive — metal — and non-conductive — textile —
components is critical. As a thread becomes more conductive and takes a bigger portion of the
conductive component, it loses the typical textile properties such as flexibility or drapability.
The structures of conductive yarn could be categorized into three classes:
- Metal-wrapped yarn is a composite of metal and yarn. A conductive yarn mainly consists of a
strand of non-conductive yarn wrapped with one or more metal wires
(See Figure 2a). - For metal-filled yarns, a fine metal wire serves as a core covered by non-conductive fibers
(See Figure 2b). Textile coverings can protect a core metal wire, helping it withstand
physical stresses and providing electrical insulation. - Metal yarn does not take a core-sheath structure. Metal fibers that are very finely drawn
replace one strand or entire strands of the yarn
(See Figure 2c). Metal fibers are prepared in forms of either filaments or staple fibers
and processed as a conventional yarn.
One or more strands of these conductive yarns are integrated into the fabric substrate to form
a textile transmission line. Successful integration creates reliable conductive traces on the
fabric while protecting the traces against repeated dimensional changes or abrasions in order to
maintain long-term conductivity. Integration methods found in the literature are divided into five
groups: woven; knitted; sewn; couched, or e-broidery; and printed structures.
The simplest way to embed conductive yarn in fabric is to weave it as one of the warp or weft
yarns. Empirically, plain weave has been preferred because its construction represents the most
elementary and simple textile structure, in which no lateral yarn movement is possible and a very
stable fabric structure is created. Consisting of interconnected loops, knitted structure is known
for its stretchability. No other textile materials can be incorporated except the conductive yarn
itself because only one continuous yarn is interlaced. Knitting requires more flexible yarns than
do any other structures because the yarn is highly curved to form a loop.
A conductive yarn can be stitched on the fabric surface to create a conductive trace. A sewn
trace forms a similar structure to the plain fabric woven with conductive yarns. It is beneficial
that a sewing line can cross over seams in apparel composition. Embroidery was previously
understood as being just for decorative purposes, but it opens much potential for smart textiles.
Conductive threads can be either embroidered or couched by traditional embroidery threads.
Embroidery using conductive threads is referred to as electronic embroidery or e-broidery. The
fabric becomes more or less rigid and offers poor flexibility.
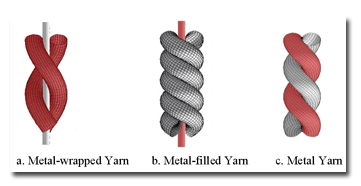
Figure 2:
Structures Of Conductive Yarns
*Conductive material is shown in red.
Interconnection
Electrical interconnection is required when a conductive path reaches to connectors (or other
conductive paths). The contact area at a junction point is critical for making a good connection.
Improper interconnection causes incomplete contact and varying contact areas that result in
non-uniform electrical resistance at the connecting points. Electrical connections are made
possible by soldering or welding, stapling, and bonding.
Soldering or welding is a process for joining two or more metals together by melting and
cooling them at the junction point
(See Figure 3a). Soldering is distinguished from welding in that the base metal is not
melted, but solder is melted and filled into the joint. Being a reliable electrical connection, the
junction point has adequate strength and electrical conductivity, which is desirable for a
permanent connection, but the hardened solder provides a bending point at which the wire can break
after repetitive bending.
Lack of flexibility can be overcome by stapling, which can be made by conductive stitches
(See Figure 3b). Stapling is highly recommended in terms of increasing flexibility at the
junction points, but increased dimensional rigidity may restrict the freedom of motion, which can
accelerate fabric tearing. With the possibility of the stitches coming loose, the stability of the
connection can be uncertain. Interconnection can be made by using conductive adhesives
(See Figure 3c). Conductive adhesives can be envisioned that are nontoxic, highly
conductive, highly durable, and moderately flexible. Bonding remains an open possibility and is the
subject of further study.
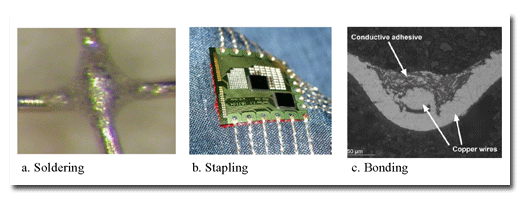
Figure 3:
Examples Of Interconnection
Connectors
Connectors between electronics and e-textiles need to be specially designed. The fastening
should be strong enough to hold the electronics and, at the same time, it should allow them to be
easily detached. Traditional forms of apparel fasteners can provide a good connection.
A two-piece gripper snap can be a good connector. One side of the snap is attached to the
e-textile and the other side to the electronic device. The major advantage of the snap is ease of
attachment and removal, but frequent connections and disconnections may weaken the strength of
interconnection between connectors and e-textiles. The size and number of snaps can limit the
connection interface and weight of electronics. Snaps are known to allow only primitive levels of
electrical connection.
For a higher-profile connector, the textile USB cable was developed
(See Figure 4). The socket has a rigid pre-mold for durability covered with a soft
over-mold for comfort. The mold system will protect the interconnection. Even more robust
connectors are proposed in a buckle shape
(See Figure 5). Physical suspension of each buckle piece will provide a more secure
connection, while it also is easily unfastened.
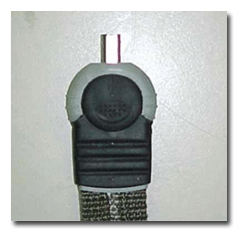
Figure 4: USB Connector
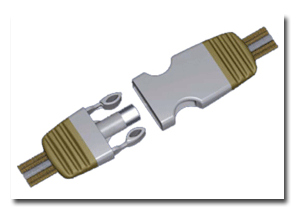
Figure 5:
Concept Of Buckle-Type Connector
Conclusion
Over the past decade, electronics have been shrinking in size and increasing in
functionality. The idea for the most wearable system is to attach technological components to the
textile in which transmission lines and connectors are embedded. Because the electronics are
attached and detached freely, they can be protected from the physical stresses of laundering. As
many different electrics can be connected to any clothing, a wearable system becomes more
versatile, and the user can change its look depending on environmental and situational changes and
individual preference.
Standardization is the biggest challenge for the industry as it commercializes the wearable
systems. It is especially critical for compatibility and connection problems. Standardization
should be done in a way that covers the multidisciplinary characteristics of an e-textile as a
textile, as an electronic, and as a computer. Another challenge is to ensure personal safety
against potential offenses from the wearable system itself or from abusive users. For example,
concerns regarding harmful effects of the electromagnetic field or leaks of confidential
information must be cleared before the clothing reaches the users.
Current advances in new materials, textile technologies, and miniaturized electronics make
wearable systems more feasible. It has been anticipated that batteries or memory storages could be
woven directly into textiles. In the future, it might be possible that people can enjoy the freedom
not to carry any electronic device, but, instead, to wear it.
Editor’s Note: Minyoung Suh is a doctoral student in the Department of Textile & Apparel
Technology & Management at North Carolina State University’s College of Textiles, Raleigh, N.C.
April 2010